Quantum Computing Definition How Its Used And Example
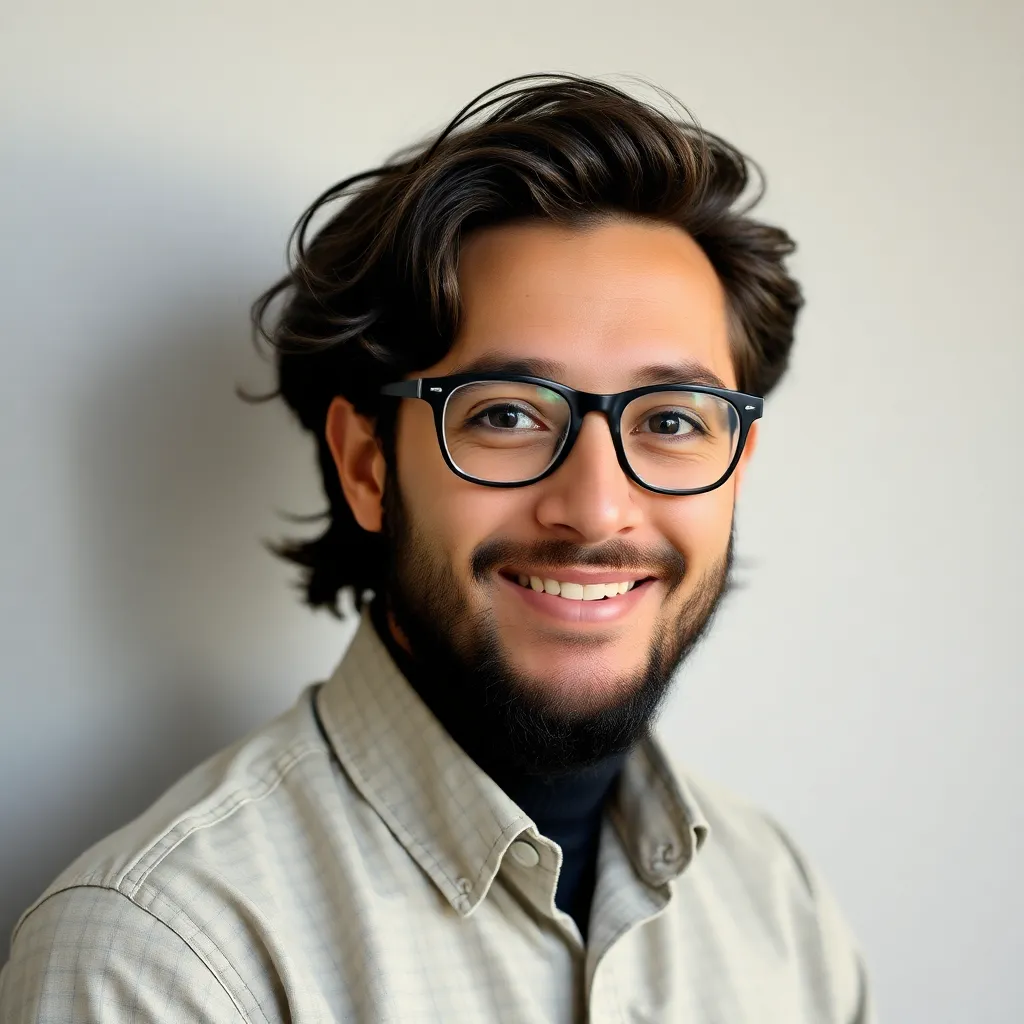
adminse
Mar 31, 2025 · 9 min read

Table of Contents
Decoding the Quantum Realm: A Deep Dive into Quantum Computing
What makes quantum computing a revolutionary leap in technological advancement?
Quantum computing is poised to redefine the boundaries of computational power, unlocking solutions to problems currently intractable for even the most powerful classical computers.
Editor’s Note: This article on quantum computing was published today, offering the latest insights into this rapidly evolving field.
Why Quantum Computing Matters
The digital age, powered by classical computers, is reaching its computational limits. Many complex problems in fields like medicine, materials science, finance, and artificial intelligence require processing power far exceeding the capabilities of even the most advanced silicon-based computers. Quantum computing offers a radical solution. By leveraging the principles of quantum mechanics, it promises to solve these currently intractable problems at unprecedented speeds, ushering in a new era of technological advancement. The potential impact spans diverse sectors, from designing novel drugs and materials to developing more efficient financial models and accelerating artificial intelligence research.
Overview of the Article
This article delves into the core concepts of quantum computing, explaining how it differs from classical computing and exploring its diverse applications. Readers will gain a foundational understanding of quantum phenomena, learn about different types of quantum computers, and discover the practical uses and limitations of this emerging technology. The article also examines the potential impact of quantum computing on various industries and addresses frequently asked questions about this revolutionary field.
Research and Effort Behind the Insights
The information presented here is based on extensive research from leading academic journals, industry reports, and contributions from experts in the field of quantum computing. Data from reputable sources has been analyzed to ensure accuracy and provide a comprehensive overview of this complex topic.
Key Takeaways
Key Insight | Description |
---|---|
Quantum vs. Classical Computing | Fundamental differences in how information is processed and stored. |
Quantum Phenomena: Superposition & Entanglement | Core principles enabling quantum computation's superior processing power. |
Types of Quantum Computers | Overview of different technologies used to build quantum computers, including superconducting, trapped ion, and photonic approaches. |
Applications of Quantum Computing | Exploration of real-world applications across various sectors, such as drug discovery, materials science, finance, and artificial intelligence. |
Challenges and Limitations | Discussion of hurdles facing the widespread adoption of quantum computing, including qubit coherence, error correction, and scalability issues. |
The Future of Quantum Computing | Speculation on the future trajectory of quantum computing, including potential breakthroughs and their impact on society and the economy. |
Let’s dive deeper into the key aspects of quantum computing, starting with its foundational principles and real-world applications.
Exploring the Key Aspects of Quantum Computing
-
Quantum Fundamentals: At the heart of quantum computing lies quantum mechanics, a branch of physics governing the behavior of matter at the atomic and subatomic levels. Unlike classical bits representing 0 or 1, quantum bits, or qubits, leverage superposition – the ability to exist in multiple states (0, 1, or a combination thereof) simultaneously. This allows quantum computers to explore many possibilities concurrently, exponentially increasing their processing power compared to classical computers. Another crucial principle is entanglement, where two or more qubits become linked, their fates intertwined regardless of the distance separating them. Manipulating entangled qubits allows for complex computations not possible with classical systems.
-
Types of Quantum Computers: Several technological approaches are being pursued to build practical quantum computers. Superconducting qubits, utilizing the properties of superconductors at extremely low temperatures, are a leading contender. Trapped ion quantum computers use individual ions trapped and manipulated using electromagnetic fields. Photonic quantum computers leverage photons (particles of light) as qubits. Each approach has its advantages and disadvantages concerning scalability, coherence times (how long qubits maintain their quantum state), and error rates.
-
Quantum Algorithms: To harness the power of quantum computers, specialized algorithms are needed. Unlike classical algorithms, quantum algorithms exploit superposition and entanglement to achieve exponential speedups for specific problems. Shor's algorithm, for instance, can factor large numbers exponentially faster than the best-known classical algorithms, posing a significant threat to current encryption methods. Grover's algorithm offers a quadratic speedup for searching unsorted databases. Developing new and more efficient quantum algorithms remains a crucial area of research.
-
Applications and Use Cases: The potential applications of quantum computing are vast and transformative. In drug discovery, quantum computers can simulate molecular interactions with unprecedented accuracy, leading to faster development of new drugs and therapies. Materials science benefits from the ability to simulate the properties of novel materials, leading to the creation of stronger, lighter, and more efficient materials. In finance, quantum computing can optimize portfolios, manage risk, and develop more sophisticated trading strategies. Artificial intelligence can be accelerated, leading to advancements in machine learning and deep learning. Quantum computing also has implications for cryptography, with the potential to break existing encryption schemes and the need for development of quantum-resistant cryptography.
-
Challenges and Limitations: Despite its immense potential, quantum computing faces several significant challenges. Maintaining qubit coherence for extended periods is crucial, as decoherence leads to errors in computation. Building and scaling up quantum computers with a large number of high-fidelity qubits is technologically demanding and expensive. Developing efficient error correction techniques is vital to ensuring the accuracy of quantum computations. The need for specialized hardware and software also presents significant hurdles for widespread adoption.
-
The Future of Quantum Computing: The field is rapidly evolving, with continuous advancements in qubit technology, algorithm development, and error correction. While widespread availability of fault-tolerant quantum computers is still years away, significant progress is being made. Collaboration between academia, industry, and governments is crucial to accelerate this progress. The long-term impact of quantum computing is likely to be profound, reshaping various industries and revolutionizing scientific discovery.
Closing Insights
Quantum computing is not merely a technological advancement; it represents a paradigm shift in computation. Its ability to tackle problems currently beyond the reach of classical computers promises transformative breakthroughs across numerous fields. While challenges remain, the ongoing research and development efforts are paving the way for a future where quantum computers will become indispensable tools for scientific discovery, technological innovation, and societal progress. The potential impact extends far beyond individual industries; it encompasses a fundamental reshaping of how we approach complex problems and unlock new levels of understanding.
Exploring the Connection Between Error Correction and Quantum Computing
Error correction is paramount in quantum computing because qubits are extremely susceptible to noise and decoherence. Unlike classical bits, qubits can lose their quantum properties easily due to interactions with the environment. This leads to errors in computation, rendering the results unreliable. Quantum error correction techniques aim to detect and correct these errors, ensuring the integrity of quantum computations. This involves encoding information across multiple qubits in a way that errors can be identified and corrected without directly measuring the qubits, preserving their quantum states. The complexity of quantum error correction is significant, requiring substantial overhead in terms of the number of qubits needed for encoding and correction. The development of robust and efficient quantum error correction codes is crucial for building large-scale, fault-tolerant quantum computers. Without reliable error correction, the practical applications of quantum computing will be severely limited.
Further Analysis of Error Correction
Aspect | Description |
---|---|
Types of Quantum Error Codes | Different approaches to encoding information across multiple qubits, including surface codes, stabilizer codes, and topological codes. |
Decoherence and Noise | The primary sources of errors in quantum computation, arising from interactions between qubits and their environment. |
Error Detection and Correction | Methods for identifying and correcting errors without destroying the quantum states of the qubits. |
Fault Tolerance | Designing quantum computers that can function reliably even in the presence of errors. |
Overhead and Scalability | The increased resource requirements (number of qubits) associated with implementing error correction, impacting the scalability of quantum computers |
FAQ Section
-
What is the difference between a classical computer and a quantum computer? Classical computers use bits representing 0 or 1, while quantum computers use qubits that can exist in superposition (0, 1, or a combination). This allows quantum computers to explore many possibilities concurrently.
-
When will quantum computers be widely available? While significant progress is being made, widespread availability of fault-tolerant quantum computers is still several years away. Near-term applications are emerging, but universal quantum computers are still under development.
-
What are the potential risks of quantum computing? One major risk is the potential to break current encryption methods, requiring the development of quantum-resistant cryptography. Ethical considerations surrounding the immense computational power of quantum computers also need careful consideration.
-
How much does a quantum computer cost? Currently, building and maintaining quantum computers is extremely expensive, limiting their accessibility to large research institutions and corporations.
-
What programming languages are used for quantum computing? Specialized quantum programming languages like Qiskit (IBM), Cirq (Google), and others are being developed. These languages allow programmers to write algorithms that leverage the unique features of quantum computers.
-
What are the ethical implications of quantum computing? The immense computational power of quantum computers raises ethical concerns, particularly regarding potential misuse in areas like cryptography, surveillance, and autonomous weapons systems.
Practical Tips
-
Learn the Fundamentals: Start by gaining a basic understanding of quantum mechanics and the core principles of quantum computing.
-
Explore Quantum Programming: Learn a quantum programming language to begin experimenting with quantum algorithms.
-
Follow Industry Developments: Keep up-to-date with the latest advancements in quantum computing by following research papers, industry news, and conferences.
-
Engage in Online Courses: Many online resources and courses provide education and training in quantum computing.
-
Network with Experts: Connect with other professionals interested in quantum computing to exchange ideas and collaborate on projects.
-
Support Research and Development: Advocate for increased investment in research and development of quantum computing technologies.
-
Consider Ethical Implications: Actively engage in discussions and debates about the ethical implications of quantum computing.
-
Identify Potential Applications: Explore how quantum computing can address challenges in your own field or area of interest.
Final Conclusion
Quantum computing stands as a beacon of technological innovation, promising to revolutionize computation and unlock solutions to currently intractable problems. While challenges remain in scaling and error correction, the rapid progress in the field signals a future where quantum computers will play a crucial role in scientific discovery, technological advancement, and societal progress. The journey into the quantum realm has only just begun, and the discoveries and insights that await are likely to reshape our world in profound ways. Further exploration and engagement with this transformative technology are not only beneficial but essential for shaping its future and harnessing its potential responsibly.
Latest Posts
Related Post
Thank you for visiting our website which covers about Quantum Computing Definition How Its Used And Example . We hope the information provided has been useful to you. Feel free to contact us if you have any questions or need further assistance. See you next time and don't miss to bookmark.